The History of the Universe in
40 Minutes:
From Big Bang to Heat Death
Michael Bleiweiss
Presented to the Ethical Society of Boston
4 June 2006
Updated 10 August 2019
0. Abstract
13.7 billion years ago, the Universe was formed in the great Big
Bang explosion. This led to the formation of galaxies, stars,
and planets, including our own little earth. This lecture
reviews our knowledge of this history, obtained through
centuries of observation and physics theory.
Michael Bleiweiss is a physicist, engineer, and computer
professional who has been interested in astronomy since
childhood. His favorite places to go were the dinosaur halls and
the planetarium in the New York Museum of Natural History.
I. Opening Words
This quotation is from The Hitchhiker's Guide to the Galaxy by Douglas Adams.
"Space is big. Really big. You just won't believe how vastly hugely
mind-bogglingly big it is. I mean, you may think it's a long way down
the road to he chemist, but that's just peanuts to space."
II. Introduction
In this talk, I will attempt to cover the entire history of the Universe
from the Big Bang to Heat Death. Throughout this discussion, I have
separated out the more technical background information as footnotes.
Before I begin, I need to give you a quick math lesson. Don't worry,
this is all stuff you've already seen back in school. In order to
describe the history of the Universe, we will need to use some very
small and some very large numbers. Physicists do this using Scientific
Notation. In Scientific Notation, numbers are represented as powers of
10. In a power of ten, a number is written as 10{x}. That x value is
called the exponent, where the number in the exponent represents the number of zeros to
put after the "1". For example:
100 = 1 followed by no zeros = 1
101 = 1 followed by one zero = 10
and so on.
For numbers less than one, a negative exponent is used. This represents
the fraction 1/10 to the power. For example:
and so on.
I also need to mention the units that are used to describe distance in
the Universe. The primary unit is called a light year, which is equal to
the distance that light travels in one year. This comes to 5.88 trillion
(5.88 x 1012) miles or 9.46 trillion (9.46 x
1012) kilometers). You might also hear of a
unit called a parsec, which is equal to 3.26 light years
1.
Finally, you will hear references to temperatures in Kelvins. A Kelvin
is defined as a temperature scale with degrees the same size as the
Centigrade scale (= (°F - 32) * 5/8)
and starting at absolute zero, which equals -273°C (-459°F).
The events detailed in this talk were derived from a combination of
astronomical observations, calculations based on experiments in particle
physics and nuclear physics, and the theory of General Relativity. This
has led to a model that is consistent with observations in both fields
-- the very large and the very small. That said, many of the fine
details are still under contention. Because of time constraints, I will
skip over details on the history of these discoveries and calculations.
III. The Big Bang
Current research indicates that the Universe began 13.7 billion years
ago (13.7 x 109 in scientific notation) in a
cataclysmic event called the Big Bang. It is not known what existed
beforehand or why the event occurred when it did
2. Also, we are only able
to calculate back to a certain point in time before which our knowledge
of physics breaks down.
In the beginning, the Universe was contained in a near single point of
near infinite density in a form called a singularity.
At 10-50 seconds, the Universe was at a
temperature of about 1035 K. At this point, all four known
physical forces were all merged into one unified force
3. These forces were: the
strong (nuclear) force, electromagnetic forces, the weak interaction (which
controls the decay of neutrons and muons), and gravity.
At about 10-42 seconds, the Universe had
cooled to 1032 K and gravity "separated"
out from the other forces. This is known as "breaking of the
symmetry."
At about 10-37 seconds, it entered a supercooled state, that is, a condition where it would normally have
undergone a quantum mechanical phase transition, but couldn't. This
created a "false vacuum" -- an energy state higher than the true vacuum
of normal space. The energy fields in this false vacuum then caused the
Universe to experience a period of extremely rapid expansion called
inflation, during which it grew by a factor of
1030 -- from 10-30
meters to 1 meter in a period of 10-35
seconds 4, 5. This
expansion caused the energy density of the fields to drop until space
underwent the phase transition into a true vacuum. The energy released
by this phase transition then created all of the particles in the
Universe.
However, in order for stars and galaxies to form, we need to have some
variations in density on smaller scales so that concentrations of matter
can collect under the pull of gravity. It turns out that these were
caused by random variations in the rate of inflation that caused it to
end at slightly different times in different locations. Where the
inflation ended later, matter was slightly denser than where it ended
earlier. These differences had a magnitude of only 1 part in 10,000, but
that was sufficient to create the complex structures that we observe
today.
Inflation has an intriguing implication. The portion of the Universe
that we can observe is limited by the distance that light can travel in
the time it has existed (about 10 - 20 billion light years). It is
likely that, prior to the inflationary period, the Universe was about
the size that light would have traveled up to that time. The rapid
expansion during inflation implies that the entire Universe might
actually be up to 1023 times larger than
what we can see!
There is an alternate model where inflation continues indefinitely. In this
scenario, each time the energy density in a local region drops sufficiently, a
local universe is created. Here, our Universe might be one of an infinite number
in a hyper-universe.
Then, at 10-34 seconds, when the Universe cooled
to about 1027 K, the strong force separated from
the electro-weak force. At this point, subatomic particles were able to
form, but it was still much too hot for protons and neutrons to exist.
All matter existed as a "soup" of quarks
6, leptons (electrons, muons, taus, and
neutrinos), and photons (radiation).
At 10-10 seconds, at a temperature of
1015 K, the weak and electromagnetic forces
separated from each other, so that all four forces were now distinct.
By 10-6 seconds, at a temperature of
1013 K, the Universe was finally cool enough
for the quarks to start "condensing" into protons and neutrons.
However, the overwhelming bulk of the mass of the Universe was in the
form of radiation (as photons). There were also large amounts of other
particles including electrons, positrons, neutrinos, and anti-neutrinos.
At 0.1 second after the Big Bang, when the Universe was at 31.5 billion K,
the density of the Universe dropped sufficiently for neutrinos to stop
interacting strongly with other particles
7 and start traveling freely. Most of
these neutrinos are probably still around, but their energy is so low
(equivalent to a temperature of 1.95 K) that they cannot be detected.
On the other hand, the remaining particles were still interacting with
each other quite vigorously, forming, annihilating, and being converted
into each other.
By one second, the temperature fell to 10 billion K. At this
temperature, the energy 8
becomes too low for electron-positron pairs to form so that their mutual
annihilation becomes dominant (this process took a while to complete --
another 30 seconds). It was also at this point that the nuclear
particles -- protons and neutrons -- became stable and achieved their
present-day ratio. However, it was still much too hot for actual nuclei
to form.
It should be pointed out that it is still not fully understood why our
Universe consists of protons and electrons and not their anti-particles.
However, it would only require 1 extra matter quark out of 300 million
total quarks to achieve the current amount of matter observed.
Nuclei could finally began to form at around 1 minute, when the
temperature dropped below 1 billion K. Only the three lightest elements
were produced in measurable quantity -- Hydrogen (75%), Helium (25%),
and Deuterium (0.03%) by mass. At this point, the evolution of the
Universe begins to slow down.
By 300,000 years, the Universe had expanded to 90 million light years
and cooled enough (4,000 K) for the atomic nuclei and electrons to start
combining into neutral atoms. This meant that the radiation (photons)
would now stop interacting with the sub-atomic particles and be able to
travel freely through space. This is referred to as decoupling.
This process lasted until the Universe was about 1 million years old.
The remnants of this are seen in the 2.7 K microwave background
radiation that is observed to come from all directions in space
9. The reason that the apparent
temperature has dropped is because as the Universe expands, the
radiation gets "stretched," making the wavelength longer, which
then corresponds to lower frequencies and energy
10. An alternate way of looking
at it is that the Universe cools as it expands. This radiation
is almost completely uniform in all directions. Recent, very precise,
satellite measurements have detected variations of 1 part in 100,000.
This is very important because it provides evidence of the small
variations in density that ultimately led to all of the complex
structure that now exists.
The blackbody radiation spectrum of the microwave background is
shown in Figure 1. A map of the variations in the background
temperature, as obtained by the Wilkinson Microwave Anisotropy
Probe (WMAP) satellite are shown in Figure 2. A summary of the
above discussion is shown in Figures 3.
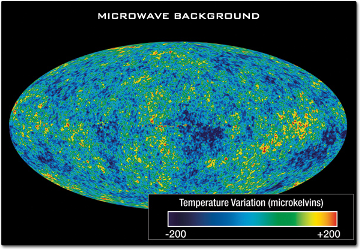
Figure 1. 2.7K Blackbody Spectrum.
Figure 2. Variations in Microwave Background
Radiation Temperature.
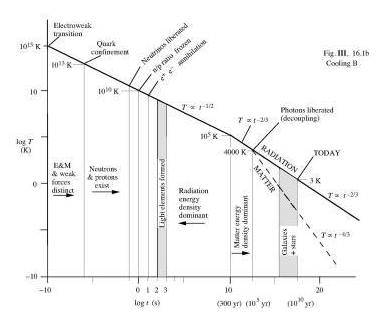
Figures 3. Evolution of the Universe. (Used
by permission of Prof. Hale Bradt - M.I.T.)
Some may ask, "Doesn't the creation of the Universe violate the law of
conservation of energy" (which states that energy can neither be created
nor destroyed). The answer to this is that the gravitational potential
energy is actually a negative energy. This is because it is "trying" to
pull the Universe back in to a point. The sum of the energy contained in
the matter (from E = mc2) plus the gravitational
potential energy add up to zero.
It should be mentioned at this point that visible matter appears to
constitute only 10% of the matter in the Universe. The remaining 90%
consists of a mysterious form of dark matter that can only be detected
due to its gravitational influence on the visible matter. Indeed, it
controls the dynamics of galaxy formation and motion. Galaxies have a
"halo" of dark matter that extends out to 10 times their visible extent.
As mentioned previously, there were small variations in the density of
matter after inflation. Once decoupling occurred, the attractive force
of gravity became greater than the repulsive force due to the pressure
of the photons bumping into everything. Therefore, the slightly denser
regions started to attract surrounding material into them. These density
variations occurred at two scales. The first was at 100,000 times the
mass of the sun (referred to as solar mass), which happens to be
the mass of a typical globular cluster (clusters of stars that orbit
the galaxies). The second was at 1012 solar
masses, which correspond to the sizes of large galaxies and small
galactic clusters.
This collapse was not uniform or monotonic. Irregularities in the
distribution and velocity of matter would have caused the collapsing
clouds to be flattened. Interactions between the gas molecules would
have induced turbulence, which would have caused heating. This, in turn,
would have caused forming clouds to break up and then reform as the
collapse continued. As this progressed, the clouds condensed into
galaxies, which then attracted to form clusters of galaxies, and
eventually, clusters of clusters (this clustering continues into the
present). The first proto-galaxies would have formed when the Universe
was about 500 million years old and coalesced over a period of about 100
million years.
There are two modes of galaxy formation. In the first, galaxies of about
1011 solar masses (about the size of our Milky
Way) form directly. This is because the physics of collapsing gas
clouds favor this size. In this case, non-uniformities in the collapse
cause the new galaxies to form as flat disks. Then, tidal forces from
nearby forming galaxies combined with gravitational forces from the dark
matter halo cause them to rotate and become spiral galaxies.
In the second mode, small, irregular galaxies form that, over time, tend
to collide and merge. Here, their respective angular momenta tend to
cancel out, causing them assume a more elliptical form 11. These collisions also caused bursts
of new star formation as their constituent gas clouds collided,
creating regions of high density. Typical spiral and elliptical galaxies are
shown in Figures 4 and 5.
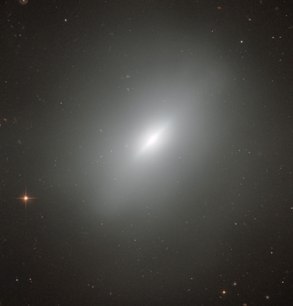
Figure 4. Spiral Galaxy Andromeda Galaxy M32.
Figure 5. Elliptical Galaxy NGC 3610.
In both cases, galaxies continue to grow into the present day by
accreting new gas from their halos and intergalactic space. Pictures of
colliding galaxies are shown in Figures 6. However, there is also an
effect counteracting this. As galaxies collide and/or interact, stars in
the outer reaches of a galaxy (known as the "halo") and gas are driven
out into intergalactic space. This can also be seen in the figure. The
force of supernova explosions also drives gas out of the galaxy proper.
NGC 4038/4039
NGC 5426/5427
Figures 6. Colliding Galaxies. (Pictures by the Hubble Space
Telescope).
A consequence of this pattern of growth, combined with the initial
density distribution, is that galaxies appear to concentrate along
filaments and sheets around the universe with large voids occupying much
of space. This is shown in Figure 7.
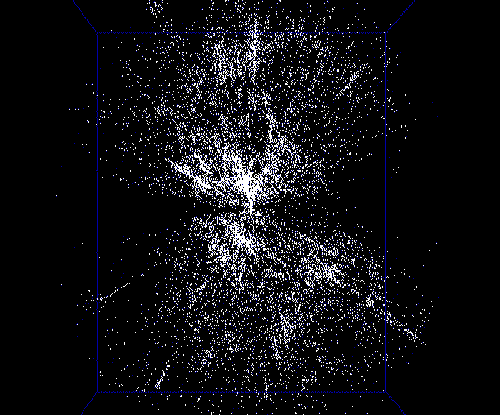
Figure 7. Distribution of Galaxies in the Local Universe (160 Mpc).
When galaxies form, their cores assemble first. In addition, as they
evolve, material tends to concentrate at their center. The resulting
extremely high density of stars and stellar remnants leads to a high
rate of collision. This, in turn, leads to the formation of a massive
black hole at the centers of most galaxies. These can range in size from
2 million to 3 billion solar masses. At first, these are very "active"
since, as material falls into them, huge amounts of energy in the form
of radiation is released. Over time, the immediate vicinity of the black
hole is depleted of material and things quiet down. An image of a black
hole at the center of the Galaxy M87 is shown as Figure 8. The galaxy
itself is shown in Figure 9. An artist's conception of a black hole is
shown in Figure 10.
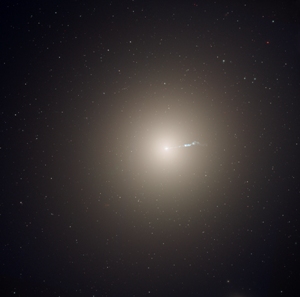
Figure 8. Image of Black Hole at Center Figure 9. M87 Galaxy.
of M87 Galaxy.
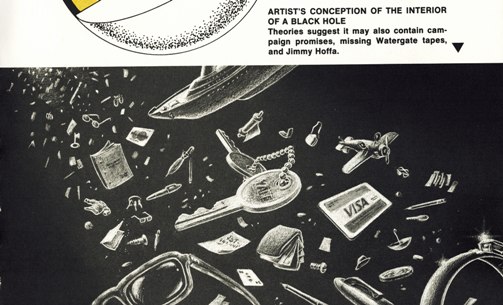
Figure 10. Artist's Conception of a Black Hole.
The continuation of this process at smaller and smaller scales within
the galactic clouds led to the formation of star-sized
aggregations, which then collapsed to form the first stars. The oldest
stars detected formed at about 2.5 billion years.
Initially, the high concentration of gas allowed many stars to form over
a short period of time. At that early time, the physics of how gas
clouds collapse 12 favored very large,
short lived stars. These went supernova, throwing most of their mass back
into space. This went on for several generations over about 100 million
years until, as the concentration of heavier elements in interstellar
space increased, the formation of smaller, longer-lived stars became
favored instead 13. The Orion Nebula -- a typical
star-forming region in our galaxy is shown in Figures 11.
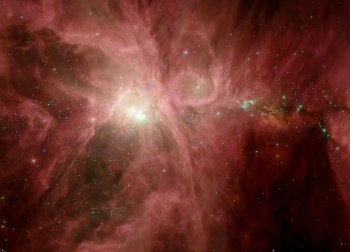
Figures 11. Orion Nebula M42.
The process quickly used up the gas in the galaxies, so that the rate of star
formation dropped off radically. Star production
seems to have peaked at about 5 billion years and has been dropping off
ever since. This is shown in Figure 12.
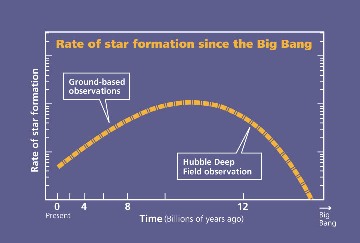
Figure 12. Rate of Star Formation vs. Age of the Universe.
Over the past couple of decades, precision measurements have
identified several thousand planets around other nearby stars (exoplanets), indicating that they are a
common phenomenon 14. That said, only a
tiny fraction appear to be capable of supporting life as we know it.
Stars generate their heat and light from nuclear reactions inside their
cores. There they "burn" hydrogen into helium through the process of
nuclear fusion. When the hydrogen is exhausted, the star starts burning
the helium into carbon, nitrogen, and oxygen. After that, heavier
elements are produced up until iron, which is the heaviest element for
which fusion will release energy rather then consume it.
The elements
heavier than iron are produced during supernova explosions, where a
massive star violently throws off most of its mass, forming a
neutron star or black hole from the remnant
15. Therefore, all of the elements
heavier than helium were produced by stars and released into space
by their nova and supernova explosions. A chart of life history
of stars as a function of their initial mass is shown in Figure 13.
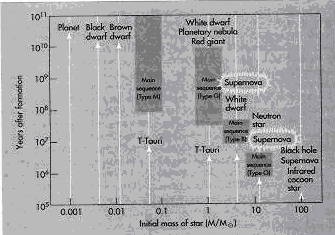
Figure
13. Fates of Stars as a Function of Mass. (from The Big Bang by Joseph Silk)
Currently, spiral galaxies are about 3% gas. Most of that is from
material thrown off by stars through their stellar winds, novae, and
supernovae. In contrast, elliptical galaxies have lost almost all of
their gas. This is because stars form sooner there and the violent
explosions expel the gas more efficiently. Consequently, star formation
there has effectively ceased and their stars are mostly older.
I will mention parenthetically here that our sun system formed when the
Universe was about 10 billion years old. As a medium-sized star, our sun
is expected to burn for another 5 billion years from now. At that time,
it will expand to be larger than the Earth's orbit. Eventually, it will
go nova and become a white dwarf.
Recent observations indicate that the expansion of the Universe may be
accelerating 16. This appears to be due to
a sort of negative gravity called dark energy 17, which appears to be due to a sort of
potential energy in the fabric of space-time. This acceleration appears
to have begun about 5 billion years ago. Up until that time, the force
of gravity was stronger than the dark energy. Then the Universe reached
a critical size and the strength of the dark energy exceeded that of
gravity. It should be noted that this is not universally accepted as
established by astronomers.
Figure 14 shows a field of galaxies from the Hubble Space Telescope Deep
Field Survey.
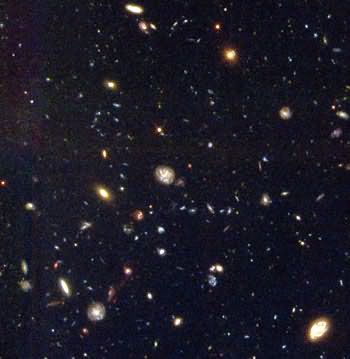
Figure 14. Picture from Hubble Deep Field Survey.
So, that brings us up to the present. The Universe is about 14 billion
years old and contains an estimated 50 billion of galaxies, each with
tens to hundreds of billions of stars. Stars are born and die. But, what
lies in our future? Even without the dark energy, the measured mass of
the Universe is only a fraction of what would be required for it to
collapse again. These two factors mean that the Universe will probably
continue to expand forever.
IV. Mid-Lecture Joke
A friend suggested that I keep my audience awake by inserting a joke in
the middle of my talk.
I wish to report the tragic death of two particle physicists. They had
gone to an Italian restaurant for lunch. One of them ordered pasta.
Without thinking, the other ordered antipasto. When their meals arrived,
they annihilated, killing them both.
V. Future Evolution
In 1996 a group of physicists at the University of Michigan performed a
calculation on the future evolution of the Universe. What follows is
highly speculative and strongly dependent on our current theoretical
understanding of particle physics. I will try to point these out as I
proceed.
We currently live in the Stelliferous Era, the period in which
stars dominate the appearance and energy budget of the Universe.
As mentioned previously, the smaller (i.e., lighter) a star, the longer
its lifetime. An average star such as our sun has a lifetime of about 10
billion years. To bring the discussion closer to home, our sun is
currently about halfway through its lifetime. In about 5 billion years,
it will have consumed most of its hydrogen and will expand into a red
giant. Current calculations indicate that in the process, it will throw
off about 30% of its mass, causing the Earth's orbit to increase enough
to move it out of range. Even so, if you have any urgent projects, you
should get to them soon.
A star with
1/10 of a solar mass will last
about 1013 years -- 1,000 times longer than
our sun. Such lower-mass stars eventually use up all of their fuel and
become white dwarves and then slowly cool into dark stellar remnants.
As stars are created and die, the amount of hydrogen in the Universe
will slowly be depleted and replaced with heavier elements. Over about
1012 years, the abundances will approach the
following: hydrogen ~20%, helium ~60%, and other elements ~20%
18. In addition, the amount of gas
available for star formation will be depleted as more and more material
becomes tied up inside of dead stars (brown dwarves 19,
white dwarves, neutron stars, and black holes). After about
1014 years, there will no longer be enough
material for new stars to form.
The lightest of the last stars to form
will live for another 1013 years, bringing the
end of the period of living stars at about
1027 years.
Again, closer to home, our galaxy and the Andromeda galaxy, which are
currently orbiting each other 2.5 million light years apart,
will eventually collide. This will probably be at some time between
6 x 1010 and 1012 years.
Once star formation has ended, the Universe will enter the Degenerate
Era which starts at 1015 years and lasts
until 1030 years. This period is characterized
by a number of phenomena.
As stars move in their orbits through a galaxy, occasionally two
brown dwarves will collide, providing enough mass for them to ignite
into small stars. This effect will be small, though, producing only a
few hundred stars per galaxy.
Most stars and stellar remnants
will merely pass near each other. Over time, their mutual gravitational
influence will tend to "slingshot" them out of the galaxy, so that
eventually over 90% of the galaxy will evaporate, leaving just the
central core region at about 1020 years.
The remaining objects will eventually drop into the central black hole
that exists at galactic cores. This will take until about year
1030.
These black holes in the clusters and superclusters of galaxies will
undergo a similar effect. Many of them will be ejected from the
supercluster and other will merge together to form even larger black holes.
It was mentioned earlier that 90% of the mass in galaxies appears to be
a mysterious form of dark matter. One model posits a new type of
particle called weakly interacting massive particles, or
WIMPs. Over time, these will be slowly absorbed by the stellar
remnants until they are depleted at about year 1025.
The captured WIMPs will tend to collide with each other and annihilate
over time. The energy from this will eventually be radiated away as
photons. The theory for this is extremely speculative.
The following is also very speculative. Many grand unified theories
assert that the proton is not stable, but will decay over long periods
of time. Currently, experiments show the proton half-life to be at least
1032 years 20,
21. Calculations also indicate that this value
is probably less than 1041 years. The paper that this analysis is based
on splits the difference and uses 1037 years.
Proton decay can take many paths, but the end products are always
photons of gamma ray energies 22,
electrons, and neutrinos. In addition, the neutrons in the atoms decay
into protons, electrons, and (anti)neutrinos.
As protons decay inside a stellar remnant, the emitted photons heat it
up, but not by much. For a typical white dwarf, proton decay would
provide about 400 watts of power, which will raise its temperature to
about 0.06 K. Over time, the star will slowly evaporate until, after
about 1038 years, it disappears completely.
Neutron stars undergo a similar evaporation. Neutron stars are not pure
neutrons. The outermost layer is ordinary matter. Therefore, the protons
that are in this outer shell begin to decay. As it loses mass, the
density of the star drops until it is converted completely into ordinary
matter. At this point, it follows a similar path as the white dwarves.
The Universe will then enter the Black Hole Era, from
1038 to 10100 years.
During this period, the black holes will also evaporate over time.
This occurs via a mechanism called Hawking Radiation
23. This is a quantum mechanical process
where pairs of virtual subatomic particles are spontaneously created at the
edge of the black hole 24. Usually, they
will almost immediately annihilate. However, sometimes one will fall
back into the black hole and the other one will escape, taking its mass
with it. The larger the black hole, the longer this process takes. A
small, star-sized black hole would disappear in about
1065 years. A typical central galactic black
hole with a mass of 1 million solar masses would take about
1083 years to completely evaporate.
The result of all of the above processes is that by about
10100 years, much of the Universe would be
made up of photons, neutrinos, electrons, positrons, and the remaining WIMPs. As expansion continues, the density of these particles decreases
and the Universe becomes colder and colder until it is a tiny fraction
of a degree above absolute zero.
This then leads to the period referred to as the Dark Era. Events
during this era become even more speculative and depend on the physical
model used.
Space is not completely empty. Despite its name, the vacuum actually
contains a sea of subatomic particles that continually come into
existence and then almost immediately disappear. This produces an energy
field throughout space. If this field is strong enough, then it might
push the Universe into another inflationary period. This would have the
effect of isolating the clusters of galaxies as they fly apart due to
the expansion of space. Depending on the strength of the field, this
could occur as early as year 1030.
If inflation does not occur and the mass in the Universe is large
enough, then large-scale variations in the structure of space may cause
particles to come together to form new black holes that would then emit
Hawking radiation. In addition, as subatomic particles such as electrons
and positions occasionally encounter each other, they will annihilate,
creating small amounts of gamma rays.
VI. Ending Joke
In the movie Hannah and her Sisters, Woody Allen's character as a young
boy is troubled about our sun going nova and destroying all life on
earth. His mother takes him to a therapist, who tells him that it won't
happen for another 5 billion years, so it's nothing that we have to
worry about.
VII. Closing Words
This song is taken from the movie "The Meaning of Life" by Monty Python
Analysis
Video Clip
Whenever life gets you down, Mrs. Brown,
And things seem hard or tough,
And people are stupid, obnoxious, or daft,
And you feel that you've had quite enough,
Just remember that you're standing on a planet that's evolving
And revolving at nine hundred miles an hour,
That's orbiting at nineteen miles a second, so it's reckoned,
A sun that is the source of all our power.
The sun and you and me and all the stars that we can see
Are moving at a million miles a day
In an outer spiral arm, at forty thousand miles an hour,
Of the galaxy we call the 'Milky Way'.
Our galaxy itself contains a hundred billion stars.
It's a hundred thousand light years side to side.
It bulges in the middle, sixteen thousand light years thick,
But out by us, it's just three thousand light years wide.
We're thirty thousand light years from galactic central point.
We go 'round every two hundred million years,
And our galaxy is only one of millions of billions
In this amazing and expanding universe.
The universe itself keeps on expanding and expanding
In all of the directions it can whiz
As fast as it can go, at the speed of light, you know,
Twelve million miles a minute, and that's the fastest speed there is.
So remember, when you're feeling very small and insecure,
How amazingly unlikely is your birth,
And pray that there's intelligent life somewhere up in space,
'Cause there's bugger all down here on Earth.
VII. References
The Inflationary Universe. Alan A. Guth.
Addison-Wesley Publishing Co, Inc. 1997.
The Extravagant Universe. Robert P. Kirshner.
Princeton University Press. 2002.
An exposition on the history of the discovery of dark energy.
The Big Bang. Joseph Silk. W.H. Freeman & Co. 2001.
A Dying Universe: The Long Term Fate and Evolution of Astrophysical Objects.
Fred C. Adams and Gregory Laughlin, University of Michigan. Reviews of Modern Physics.
Vol. 69, pg. 337-372 (April 1997).
Very Distant Supernovae Suggest that the Cosmic Expansion is Speeding Up.
Bertram Schwarzschild. Physics Today, June 1998. pg. 17-19.
Lonely Universe. Ron Cowen. Science News. V 162. 31 August 2002.
pg. 139-40.
Cosmic Revelations: Satellite Homes in on the Infant Universe. Science News. V. 163. 15 February 2003. pg. 99-100
For very brief summaries of the future history of the Universe, see:
Hubble Space Telescope Pictures and Information
Hubble Space Telescope Pictures and Information:
http://HubbleSite.org
Andy Kravtsov:
Distribution of Galaxies and Clusters.
University of Chicago
New date for initial star formation:
Steve Nadis. New Scientist 181.2435 (Feb 21, 2004): p32(4).
Images:
Spiral Galaxy: Andromeda Galaxy = M31: Adam Evans
https://upload.wikimedia.org/wikipedia/commons/9/98/Andromeda_Galaxy_%28with_h-alpha%29.jpg
Elliptical Galaxy: NGC 3610: Hubble Telescope
https://3c1703fe8d.site.internapcdn.net/newman/gfx/news/hires/2015/2-hubbleviewsa.jpg
Colliding Galaxies NGC 4038/4039: Hubble Space Telescope
https://HubbleSite.org/contents/media/images/1997/34/538-Image.html?keyword=NGC%204038
Colliding Galaxies NGC 5426/5427: Hubble Space Telescope
https://HubbleSite.org/contents/media/images/1997/34/536-Image.html?keyword=NGC%205426
M87* Galactic Black Hole - New Horizons Telescope Collaboration - NSF
https://phys.org/news/2019-04-astronomers-unveil-photo-black-hole.html
M87 Galaxy: NASA
https://www.nasa.gov/sites/default/files/thumbnails/image/m87-full_jpg.jpg
Artist's Conception of Black Hole:
Science Made Stupid. Tom Weller. Houghton Mifflin Co. Boston. 1985.
2.7K Blackbody Microwave Spectrum: The Microwave Background. Michael
Richmond.
Article: http://spiff.rit.edu/classes/phys240/lectures/cmb/cmb.html
Image: http://spiff.rit.edu/classes/phys240/lectures/cmb/firas_spectrum.jpg
Microwave Background Radiation Variation: NASA
Article: https://science.nasa.gov/ems/06_microwaves
Image:
https://smd-prod.s3.amazonaws.com/science-pink/s3fs-public/thumbnails/image/microwave-7.jpg
Evolution of Universe Graphs: Prof. Hale Bradt. Physics Dept. Massachusetts
Institute of Technology.
Orion Nebula Full: http://www.astrocruise.com/milky_way/M42_0712.htm - Philip
Perkins 12/13/2007
Orion Nebula Close up: http://spaceref.com/astronomy/the-orion-nebula.html
- NASA Spitzer Science Center 07/23/2013
Rate of Star Formation Since Big Bang: NASA & ESA
Article: https://www.SpaceTelescope.org/images/opo9832m
Image: http://cdn.SpaceTelescope.org/archives/images/screen/opo9832m.jpg
VIII. Footnotes
-
The parsec is defined as the distance required for an angle of 1" to
spread out to a separation equal to the diameter of the Earth's orbit
(184 million miles). back
-
There is an extension of String Theory that posits a "hyper-universe"
containing of a set sheets called "M-branes." The theory states that
when two of these collide, it results in a release of energy that causes
a universe to form. back
-
There are a set of theories called "Grand Unified Theories" (or GUT)
that describe the behavior of sub-atomic particles. An essential part of
these is the principle that, as particle energy increases, the forces
that we observe as separate "merge" together. A branch of thermodynamics
known as Statistical Mechanics equates temperature with the average
speed of a group of particles (usually atoms and molecules at everyday
conditions). Since speed converts to kinetic energy, particle energy can
be equated to an equivalent temperature. back
-
The energy of the false vacuum was contained in quantum fields called
Higgs Fields. These are generated by the recently discovered particle
called the Higgs boson, which is attributed with creating the mass of
all of the particles. Its estimated mass is 125 GeV, which is at the limit of
the world's largest particle accelerator at CERN in Geneva, Switzerland. back
-
Intellectual honesty requires me to state that these numbers are only
approximate. The values depend critically on the specific Grand Unified
Theory that is used in making the calculations. That said, the concept
of inflation helps to explain why the Universe is so uniform on a large
scale -- if it was approximately uniform to begin with, the rapid
expansion enhances the uniformity. back
-
A quark can be thought of as a sub-sub-atomic particle. Protons and
neutrons are each made up of 3 quarks. The name is derived from a line
in the James Joyce novel Finnegan's Wake: "Three quarks for Muster Mark."
back
-
Neutrinos interact extremely weakly with other matter. It is estimated
that a typical neutrino could travel through 3,500 light years of rock
before being stopped. back
-
When a sub-atomic particle and it's anti-particle collide, they
annihilate each other, releasing a burst of high-energy gamma ray
photons. Electrons and positrons have a mass-energy equivalent of
0.511 MeV. back
-
This radiation was discovered in 1964 by Arno Penzias and Robert Wilson
at Bell Labs when they were trying to determine the source of "noise" in
their radio telescope. It had also been predicted theoretically by a
number of physicists in the 1950s and early 1960s. Penzias' and Wilson's
measurements provided confirmation to their calculations.
back
-
For electromagnetic radiation, the energy in a photon = E =
h/2(π)(λ),
where λ = wavelength and h = Plank's Constant = 6.626 x 10-27
erg-second. back
-
This process tends to occur mostly in large clusters of galaxies where
there is a massive central galaxy. Spiral galaxies tend to form within
sparse groups. It should be noted that small irregular galaxies and
globular clusters will also form in these sparse groups. However, here
they are much less likely to collide. back
-
As a gas cloud collapses, it heats up. This increases the pressure,
which then slows the collapsing process. Hydrogen gas cools very
inefficiently. In order to lose energy, their electron must be bumped up
to a higher energy state via collisions with other atoms. They will then
emit a photon when the electron drops back down into its "ground state,"
releasing the energy out into space. It turns out that this causes the
resulting final stellar cloud to be more massive.
back
-
As more elements were released into space, more energy levels became
available to radiate energy out of the collapsing clouds. This then
allowed them to break up into smaller units and form smaller stars.
back
-
We cannot actually see planets around other stars. Rather, a
dimming of the star's light is observed as a planet transits
across its face or the star's position wobbles as the planet
orbits around it. back
-
Stars lighter than about 6 solar masses go nova when they
have exhausted all of their nuclear fuel, leaving stellar remnants lighter than
1.4 solar masses, becoming white dwarves. These are held up by the
natural fermi repulsion between electrons. Stars between 30 and 50 solar
masses throw off most of their mass in a supernova, leaving a remnant
between 1.4 and 2.2 solar masses, becoming neutron stars. Here, the
gravitational forces are so great that the electrons and protons are
forced together to become neutrons. They are then held up by the fermi
repulsion between the neutrons. Stars heavier than that produce remnants
with masses greater than 2.2 solar masses and become black holes, whose
gravity is so great that even light cannot escape. back
-
How do we know about how the Universe is expanding? This is done by
measuring the spectra of the stars in very distant galaxies. You can
think of the Universe as being the surface of a balloon that is being
blown up. Points that are further around on the balloon will move away
from us more quickly. Also, as they move away from us, the light they
emit gets "stretched," causing its wavelength to become longer, which
makes it appear redder. This is known as the red shift. We can
then tell how far away they are by making various brightness measurements
and comparing them to the known brightness of similar objects that are
closer. This process would require an entire additional lecture to
describe fully. back
-
When Albert Einstein first developed the General Theory of Relativity in
1916, it was before Edwin Hubble's discovery in the 1920s that the
Universe was expanding. Therefore, Einstein added a Cosmological
Constant as a countervailing force to gravity over very large distances
to explain why the Universe didn't collapse on itself. He later
described it as his "greatest mistake." However, this discovery shows
that his initial equation was correct, but for the wrong reason.
back
-
In astrophysics, any elements heavier than Helium are referred to as
metals and their abundance is referred to as metalicity. back
-
Brown dwarves are protostars that are too light to begin nuclear fusion,
generally < 0.08 solar masses. back
-
The tests for proton decay consist of tanks containing tens of thousands
of gallons of ultra-pure water buried deep underground where they are
shielded from external energy sources such as cosmic rays (high energy
nuclei) gamma rays. back
-
A half life is the length of time that it takes for half of the
nuclei or sub-atomic particles in a sample to decay.
back
-
Gamma rays from particle decay typically have energies of
5x105 to 5x108 eV.
An electron volt (eV) is the kinetic energy possessed by an electron
that has been accelerated through a 1 volt potential. back
-
Named after British physicist Stephen Hawking, who first advanced the theory in
1974.
back
-
This phenomenon is due to the Heisenberg Uncertainty Principle.
This states that the product of the uncertainty in a particle's energy
and lifetime must be greater than Plank's Constant (h = 6.63×10-34
J·s = 4.14×10-15 eV·s) divided by 2π. That
is ΔE x Δt >= h/2π.
The consequence of this is that a particle can spontaneously come into
existence without violating conservation of energy as long as it
disappears again quickly enough. back
TOP